Mass Spectroscopy-Introduction
UncategorizedMass spectrometry (MS) is a powerful and versatile analytical tool for obtaining information about the identity of an unknown compound, its molecular mass, its elemental composition, and in many cases, its chemical structure. Mass spectrometry can be conveniently divided into atomic, or elemental, mass spectrometry and molecular mass spectrometry. Atomic mass spectrometry is a quantitative tool that can determine nearly all the elements in the periodic table. Detection limits are often several orders of magnitude better than optical methods. On the other hand, molecular mass spectrometry is capable of providing information about the structures of inorganic, organic, and biological molecules and about the qualitative and quantitative composition of complex mixtures. We first discuss the principles that are common to all forms of mass spectrometry and the components that constitute a mass spectrometer.
Principle:
In the mass spectrometer, analyte molecules are converted to ions by applying energy to them. The ions formed are separated on the basis of their mass-to-charge ratio (m/z) and directed to a transducer that converts the number of ions (abundance) into an electrical signal. The ions of different mass-to-charge ratios are directed to the transducer sequentially by scanning or made to strike a multichannel transducer simultaneously. The ion abundance plotted against mass-to-charge ratio is called a mass spectrum.
Atomic masses:
The chemical atomic mass, or the average atomic mass, of an element in nature is given by summing the exact masses of each isotope weighted by its fractional abundance in nature. The chemical atomic mass is the type of mass of interest to chemists for most purposes. The average or chemical molecular mass of a compound is then the sum of the chemical atomic masses for the atoms appearing in the formula of the compound. Thus, the chemical molecular mass of CH4 is 12.011 + 4 * 1.008 = 16.043 Da. The atomic or molecular mass expressed without units is the mass number
One unified atomic mass unit on this scale is equal to 1/12 the mass of a neutral 126C atom. The unified atomic mass is given the symbol u. One unified mass unit is commonly termed one dalton (Da), which has become the accepted term even though it is not an official SI unit. The older term, atomic mass unit (amu), is to be discouraged since it was based on the most abundant stable isotope of oxygen 16O.
Mass to charge ratio:
The mass-to-charge ratio, m/z, of an ion is the quantity of most interest because the mass spectrometer separates ions according to this ratio. The mass-to-charge ratio of an ion is the unit less ratio of its mass number to the number of fundamental charges z on the ion. Thus, for 12C1H4+, m/z = 16.032/1 = 16.032. For 13C1H42+, m/z = 17.032/2 = 8.516.
Mass Spectrometers:
The mass spectrometer is an instrument that produces ions, separates them according to their m/z values, detects them, and plots the mass spectrum. Such instruments vary widely in size, resolution, flexibility, and cost. Their components, however, are remarkably similar.
Components of Mass Spectrometers:
In molecular mass spectrometry, samples enter the evacuated region of the mass spectrometer through the inlet system. Solids, liquids, and gases may be introduced depending on the nature of the ionization source. The purpose of the inlet system is to introduce a micro amount of sample into the ion source where the components of the sample are converted into gaseous ions by bombardment with electrons, photons, ions, or molecules. In atomic mass spectrometry, the ionization source is outside the evacuated region and also serves as the inlet. In atomic mass spectrometers, ionization is accomplished by applying thermal or electrical energy. The output of the ion source is a stream of positive (most common) or negative gaseous ions. These ions are accelerated into the mass analyzer, which then separates them according to their mass-to-charge ratios. The ions of particular m/z values are then collected and converted into an electrical signal by the ion transducer. The data handling system processes the results to produce the mass spectrum. The processing may also include comparison to known spectra, tabulation of results, and data storage.
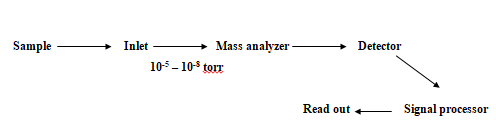
Mass analyzer:
Six type of Mass analyzer available;
- Magnetic sector
- Double-focusing
- Quadrupole
- Ion trap
- Ion cyclotron resonance
- Time-of-flight
Resolution of Mass spectrometers:

△m = mass difference between two adjacent peaks
m = the nominal mass of the first peak
A resolution of 100 means that unit mass (1 Da) can be distinguished at a nominal mass of 100.
Quadrupole Mass Analyzers:
Quadrupole analyzers are mass filters that only allow ions of a certain mass-to-charge ratio to pass. Ion motion in electric fields is the basis of separation. Rods opposite each other are connected to dc and radiofrequency (RF) voltages. With proper adjustment of the voltages, a stable path is created for ions of a certain m/z ratio to pass through the analyzer to the transducer. The mass spectrum is obtained by scanning the voltages applied to the rods. Quadrupole analyzers have relatively high throughput but relatively low resolution. Unit mass (1 Da) is the typical resolution of a quadrupole analyzer. This resolution may be sufficient in many forms of elemental mass spectrometry or in cases where a mass spectrometer serves as a detector for molecules separated by gas or liquid chromatography.
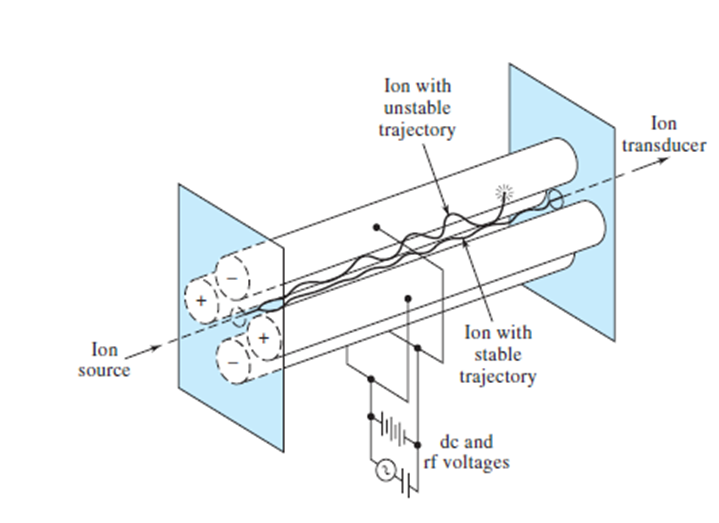
.
Quadrupole mass analyzer
Time-of-Flight Mass Analyzers:
The time-of-flight (TOF) mass spectrometer represents another approach to mass analysis. In a TOF analyzer, a packet of ions with nearly identical kinetic energies is rapidly sampled, and the ions enter a field-free region. Since the kinetic energy, KE, is ½mv2, the ion velocity v varies inversely with its mass.

The time required for the ions to travel a fixed distance to the detector is thus inversely related to the ion mass. In other words, ions with low m/z arrive at the detector more rapidly than those with high m/z. Each m/z value is then detected in sequence. Flight times are quite brief, leading to analysis times that are typically on the order of microseconds. Time-of-flight instruments are relatively simple and rugged and have nearly unlimited mass range. The TOF analyzer suffers, however, from limited resolution and sensitivity. As a result, TOF analyzers are less widely used than magnetic sector and quadrupole analyzers.
ICP – MS (Inductively Coupled Plasma-mass spectroscopy):
ICP serves as both an atomizer and an ionizer. Solution samples may be introduced by a conventional or an ultrasonic nebulizer. Solid samples can be dissolved in solution or volatized by means of a high-voltage spark or high-powered laser prior to introduction into the ICP. Ions formed in the plasma are then introduced into the mass analyzer, often a quadrupole, where they are sorted according to mass-to-charge ratio and detected.
While an ICP operates at atmospheric pressure, a mass spectrometer operates at high vacuum, typically less than 1026 torr. The interface region between the ICP and the mass spectrometer is thus critical to ensure that a substantial fraction of the ions produced are transported into the mass analyzer. The interface usually consists of two metal cones, called the sampler and the skimmer. Each cone has a small orifice (< 1 mm) to allow the ions to pass through to ion optics that then guide them into the mass analyzer. The beam introduced into the mass spectrometer has about the same ionic composition as the plasma region from which the ions are extracted.
Detection limits for quadrupole ICPMS instruments are often less than 1 ppb.
Molecular Mass Spectroscopy:
The analyte is ethyl benzene, which has a nominal molecular mass of 106 daltons (Da). To obtain this spectrum, ethyl benzene vapor was bombarded with a stream of electrons that led to the loss of an electron by the analyte and formation of the molecular ion M1, as shown by the reaction.

The charged species C6H5CH2H3. + is the molecular ion. As indicated by the dot, the molecular ion is a radical ion that has the same molecular mass as the molecule.
The collision between energetic electrons and analyte molecules usually imparts enough energy to the molecules to leave them in an excited state. Relaxation then often occurs by fragmentation of part of the molecular ions to produce ions of lower masses. For example, a major product in the case of ethyl benzene is C6H5CH2+, which results from the loss of a CH3 group. Other smaller positively charged fragments are also formed in lesser amounts.
The positive ions produced on electron impact are attracted through the slit of a mass spectrometer, where they are sorted according to their mass-to-charge ratios and displayed in the bar graph form of a mass spectrum, Mass spectrum of ethyl benzene, that the largest peak at m/z = 91, termed the base peak, has been arbitrarily assigned a value of 100. The heights of the remaining peaks are then computed as a percentage of the base-peak height.
Ion source:
The starting point for a mass spectrometric analysis is the formation of gaseous analyte ions, and the scope and the utility of a mass spectrometric method is dictated by the ionization process. The appearance of mass spectra for a given molecular species is highly dependent on the method used for ion formation.
Gas –Phase sources: the sample is first vaporized and then ionized
Desorption source: the sample in a solid or liquid state is converted directly into gaseous ions. An advantage of desorption sources is that they are applicable to nonvolatile and thermally unstable samples.
Gas – phase:
Electron impact (EI) – molecules are bombarded with a high-energy beam of electrons. This produces positive ions, negative ions, and neutral species. The positive ions are directed toward the analyzer by electrostatic repulsion.
In EI, the electron beam is so energetic that many fragments are produced. These fragments, however, are very useful in identifying the molecular species entering the mass spectrometer. Mass spectra for many libraries of MS data have been collected using EI sources.
Instrumentation:
Inlet Systems:
The major types of inlets can be classified as batch inlets, direct probe inlets, chromatographic inlets, and electrophoretic inlets. The batch inlet is the most common for introducing liquids and gases.
The conventional (and simplest) inlet system is the batch type in which the sample is volatilized externally and then allowed to leak into the evacuated ionization region. Liquids and gases can be introduced in this way.
Solids can be placed on the tip of a probe, inserted into the vacuum chamber, and evaporated or sublimed by heating. Nonvolatile liquids can be introduced through special controlled-flow inlets, or they can be desorbed from a surface on which they are coated as a thin film. In general, samples for molecular mass spectrometry must be pure because the fragmentation that occurs causes the mass spectrum of mixtures to be difficult to interpret. Gas chromatography is an ideal way to introduce mixtures because the components are separated from the mixture by the chromatograph prior to introduction to the mass spectrometer. The combination of gas chromatography and mass spectrometry is often called GC/MS.
High-performance liquid chromatography and capillary electrophoresis can also be coupled with a mass spectrometer through the use of specialized interfaces.
Mass analyzer:
The quadrupole mass analyzer is commonly used with GC/MS systems. Higher resolution spectrometers (magnetic sector, double-focusing, time-of-flight, Fourier transform) are often used when fragmentation patterns are to be analyzed for structural or identification purposes.
Tandem mass spectrometry, also called mass spectrometry-mass spectrometry (MS/MS), is a technique that allows the mass spectrum of a preselected or fragmented ion to be obtained. With a tandem mass spectrometer, an ionization source produces molecular ions and fragment ions. These are then the input to the first mass analyzer, which selects a particular ion (the precursor ion) and sends it to the interaction cell. In the interaction cell, the precursor ion can decompose spontaneously, react with a collision gas, or interact with an intense laser beam to produce fragments, or product ions. These ions are then mass analyzed by the second mass analyzer and detected by the ion detector.
Tandem mass spectrometers can produce a variety of different spectra. roduction spectra are obtained by scanning mass analyzer 2 while mass analyzer 1 is held constant to act as a mass selector for the precursor ion. A precursor-ion spectrum can be obtained by scanning mass analyzer 1 and selecting a given product ion with mass analyzer 2. If both mass analyzers are scanned with a small offset in mass between them, a neutral loss spectrum can be obtained. A neutral loss spectrum might be used, for example, to identify the m/z values of all ions losing a common molecule, such as water. Finally, a complete three-dimensional MS/MS spectrum can be acquired by recording a product ion spectrum for each selected precursor ion, that is, by scanning mass analyzer 2 for various settings of mass
analyzer 1.
Tandem mass spectrometry can produce an enormous amount of information and has proven useful in structural elucidation as well as in the analysis of mixtures. Conventional mass spectrometry of mixtures usually requires chromatographic or electrophoretic separation to present a single compound at a time to the mass spectrometer.
Application:
- Elucidation of the structure of organic and biological molecules
- Determination of the molecular mass of peptides, proteins, and oligonucleotides
- Identification of components in thin-layer and paper chromatograms
- Determination of amino acid sequences in sample of polypeptides and proteins
- Detection and identification of species separated by chromatography and capillary
- electrophoresis
- Identification of drugs of abuse and metabolites of drugs of abuse in blood, urine, and saliva
- Monitoring gases in patient’s breath during surgery
- Testing for the presence of drugs in blood in thoroughbred race horses and in Olympic athletes
- Dating archaeological specimens
- Analysis of aerosol particles
- Determination of pesticide residues in food
- Monitoring volatile organic species in water supplies
- Analysis of mixture
- Identification of pure compounds
- Quantitative analysis.
(Reference: Fundamentals-of-Analytical-Chemistry-by-Douglas-A.-Skoog-9th-Ed page No : 802-817)